
Overcoming Failure
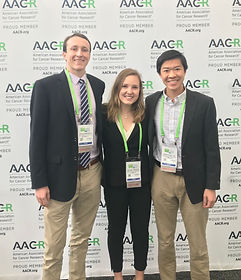
I attended the American Association for Cancer Research (AACR) Conference in March 2019 along with other USC Biochemistry and Biomedical Engineering students
Fall semester of my sophomore year was when my time at USC significantly changed; it began when I first inquired my BMEN 389: Stem Cell Engineering professor, Dr. Ehsan Jabbarzadeh, about a research position in his lab. For as long as I can remember, I have always had a passion for scientific inquiries and discoveries and knew that I wanted to pursue research during my academic career. After briefly learning about relatively recent discoveries made in stem cells research and the amount of information yet to be discovered in my introductory biomedical engineering classes (BMEN 101 and BMEN 211), I decided to take BMEN 389: Stem Cell Engineering, taught by Dr. Ehsan Jabbarzadeh. In BMEN 389, we delved more comprehensively into research regarding the use of stem cells in regenerative medicine, including some of the research that was actively being performed in his lab group. He had his postdoctoral fellow, Dr. Maria Yanez, give a presentation to the class on her PhD dissertation regarding the modification and use of a regular inkjet printer to print a bilayer skin graft, and his graduate student, Wesley Taylor, discuss and demonstrate basic laboratory techniques and assays. The accumulation of the information learned in the class resulted in a presentation outlining a recently-published paper in the field of stem cell engineering
and a group project aimed at identifying a novel regenerative medicine solution for a substantial problem in biomedical sciences. The solution and problem were then extensively discussed in a mock National Institute of Health (NIH) R21 grant. After observing the Jabbarzadeh lab members teach and participate in two class projects on regenerative medicine, I knew that I wanted to research in the Jabbarzadeh Lab under the mentorship of Dr. Jabbarzdeh.
The development of a thermoresponsive hydrogel for modulation of cell behavior was the major research project I worked on in the Jabbarzadeh Lab at USC. The goal of this project was to develop a novel non-cytotoxic platform for inducing slight mechanical stress on the cellular membrane in order to either increase the permeability of the membrane to compounds or induce mechanically-significant differentiation of stem cells, namely mesenchymal stem cells (MSCs). Under the direct mentorship of Dr. Maria Yanez, the project has two major objectives: development and demonstration of the platform’s ability in inducing mechanical stress and determination of the effects that mechanical stress has on cellular behavior. I was awarded the Science Undergraduate Research Fellowship (SURF) grant through the South Carolina Honors college totaling $4500 and the Magellan Scholar award totaling $2750 in stipends.
To accomplish the first objective of the project, the development of the platform, I had to find a material that has to be biocompatible and is responsive where the degree of response to effect can be easily manipulated. My first attempt at completing this

Poster Presentation at the American Association for Cancer Research (AACR) Conference in March 2019
objective was to depend solely on the swelling properties of common hydrogels (i.e. Polyethylene glycol). Amidst developing the polyethylene glycol-based hydrogel, I realized that the issue with relying on hydrogel swelling on inducing mechanical stress is that the swelling is not easily controllable and would fail in producing the desired effect. Due to this failure, I had to learn from the shortcomings of my first attempt, discover the underlying issues that caused the failure, and apply this knowledge to derive new solutions; this new solution was through polymer science, or more specifically, a class of materials, called smart materials. Smart materials are materials that alter its mechanical properties, usually in the form of morphological changes, in response to an environmental change (i.g. Temperature, pH, magnetic field). The smart material that I found suitable was a PNIPAM-based hydrogel which exhibits significant changes in swelling properties between room and physiological temperatures depending on the hydrogel formulations. The adaptability of PNIPAM allows for a more versatile platform that is far better at creating the desired effect.
In order to accomplish the second objective of the project, the implementation of cells onto the platform, I had to find methods to isolate single cells into the microwells and somehow visualize the mechanical stress exerted on the overall cell, or more specifically, the cellular membrane. At first, I attempted the method of centrifugation to draw individual cells into the cell-sized microwells, but the issue was that some cells remained on top of the hydrogel surface without being deposited into the microwells due to the elasticity of the hydrogel. Analyzing the result of this method, I sought a more dynamic approach through a fluid mechanics solution. Through this approach, I found that a more efficient method for single-cell isolation was to utilized microfluidic concepts, where cell media is fluidically manipulated over the PNIPAM microwells. Turbulent flow above individual microwell drags and isolate individual cells in each microwell. By assuming a fluid mechanics solution for this issue, I was able to find a more effective method.
After my first semester of joining the Jabbarzadeh research group, I was enrolled in a core biomedical engineering class, BMEN 290: Thermodynamics of Biomolecular Systems. In this class, we examined several thermodynamic concepts and mathematic relationships for thermodynamic variables. After taking math-heavy science classes such as MATH 241, PHYS 211, and PHYS 212, I learned the skill of first determining the spatial concepts that dictate specific scientific scenarios and then adapting mathematic functions to fit and further explain those concepts. That is, to have the mathematical equations building off of the underlying, fundamental concepts. Because of this, I went into BMEN 290 with the premise that I must first attempt to spatially understand the concepts preceding the event then adjust thermodynamic functions to fit those concepts, similar to what I had depended on in my other core science classes. But as I soon realized, this mentality was not effective in the field of thermodynamics. With this strategy, I was failing to comprehend the basic concepts of thermodynamics which led me to be behind in the class as several concepts in the class build off of the previous concepts. Understanding my insufficiencies in learning methodology for understanding thermodynamics, I knew that something had to change in order for me to succeed in the class and successfully comprehend thermodynamics, a fundamental subject on which several scientific fields depend on. For this, I had to first understand the underlying reasons why my current methods were insufficient. I knew that I was attempting to conceptually understand the thermodynamic scenarios before applying and solving mathematical relationships. Numerical concepts in thermodynamics are usually extremely abstract or arbitrarily defined, so spatial concepts are not easily understood. Instead, I needed to understand the mathematical thermodynamic functions first in order to input thermodynamic variables and computing unknowns that dictate what is conceptually occurring in the thermodynamic system. By applying this strategy, I was able to succeed in BMEN 290 and efficiently learn thermodynamics.
Within both my research in the Jabbarzadeh lab and BMEN 290, I learned to treat failures not only as steps closer to the final solution but also as vital learning experiences from which I utilize and tune future methodology in order optimize the final solution. Uniquely, my research project taught me to discover various other research techniques similar or even just small modifications to the techniques I have attempted in order to find the perfect solution. On the other hand, my learning experiences in BMEN 290 showed me that sometimes the correct solution is very different from what I was originally thought. Nevertheless, both of my experiences demonstrated the uniqueness of solutions and the importance of overcoming and learning from failures. In the research and engineering fields, documentation of failures is extremely critical; the documentation justifies the implementation of strategies and shows other researchers/engineers what methods have already been performed and failed so that repetition of failures can be prevented and effective solutions can be optimized.
The development of a thermoresponsive hydrogel for modulation of cell behavior was the major research project I worked on in the Jabbarzadeh Lab at USC. The goal of this project was to develop a novel non-cytotoxic platform for inducing slight mechanical stress on the cellular membrane in order to either increase the permeability of the membrane to compounds or induce mechanically-significant differentiation of stem cells, namely mesenchymal stem cells (MSCs). Under the direct mentorship of Dr. Maria Yanez, the project has two major objectives: development and demonstration of the platform’s ability in inducing mechanical stress and determination of the effects that mechanical stress has on cellular behavior. I was awarded the Science Undergraduate Research Fellowship (SURF) grant through the South Carolina Honors college totaling $4500 and the Magellan Scholar award totaling $2750 in stipends.
To accomplish the first objective of the project, the development of the platform, I had to find a material that has to be biocompatible and is responsive where the degree of response to effect can be easily manipulated. My first attempt at completing this objective was to depend solely on the swelling properties of common hydrogels (i.e. Polyethylene glycol). Amidst developing the polyethylene glycol-based hydrogel, I realized that the issue with relying on hydrogel swelling on inducing mechanical stress is that the swelling is not easily controllable and would fail in producing the desired effect. Due to this failure, I had to learn from the shortcomings of my first attempt, discover the underlying issues that caused the failure, and apply this knowledge to derive new solutions; this new solution was through polymer science, or more specifically, a class of materials, called smart materials. Smart materials are materials that alter its mechanical properties, usually in the form of morphological changes, in response to an environmental change (i.g. Temperature, pH, magnetic field). The smart material that I found suitable was a PNIPAM-based hydrogel which exhibits significant changes in swelling properties between room and physiological temperatures depending on the hydrogel formulations. The adaptability of PNIPAM allows for a more versatile platform that is far better at creating the desired effect.
In order to accomplish the second objective of the project, the implementation of cells onto the platform, I had to find methods to isolate single cells into the microwells and somehow visualize the mechanical stress exerted on the overall cell, or more specifically, the cellular membrane. At first, I attempted the method of centrifugation to draw individual cells into the cell-sized microwells, but the issue was that some cells remained on top of the hydrogel surface without being deposited into the microwells due to the elasticity of the hydrogel. Analyzing the result of this method, I sought a more dynamic approach through a fluid mechanics solution. Through this approach, I found that a more efficient method for single-cell isolation was to utilized microfluidic concepts, where cell media is fluidically manipulated over the PNIPAM microwells. Turbulent flow above individual microwell drags and isolate individual cells in each microwell. By assuming a fluid mechanics solution for this issue, I was able to find a more effective method.
After my first semester of joining the Jabbarzadeh research group, I was enrolled in a core biomedical engineering class, BMEN 290: Thermodynamics of Biomolecular Systems. In this class, we examined several thermodynamic concepts and mathematic relationships for thermodynamic variables. After taking math-heavy science classes such as MATH 241, PHYS 211, and PHYS 212, I learned the skill of first determining the spatial concepts that dictate specific scientific scenarios and then adapting mathematic functions to fit and further explain those concepts. That is, to have the mathematical equations building off of the underlying, fundamental concepts. Because of this, I went into BMEN 290 with the premise that I must first attempt to spatially understand the concepts preceding the event then adjust thermodynamic functions to fit those concepts, similar to what I had depended on in my other core science classes. But as I soon realized, this mentality was not effective in the field of thermodynamics. With this strategy, I was failing to comprehend the basic concepts of thermodynamics which led me to be behind in the class as several concepts in the class build off of the previous concepts. Understanding my insufficiencies in learning methodology for understanding thermodynamics, I knew that something had to change in order for me to succeed in the class and successfully comprehend thermodynamics, a fundamental subject on which several scientific fields depend on. For this, I had to first understand the underlying reasons why my current methods were insufficient. I knew that I was attempting to conceptually understand the thermodynamic scenarios before applying and solving mathematical relationships. Numerical concepts in thermodynamics are usually extremely abstract or arbitrarily defined, so spatial concepts are not easily understood. Instead, I needed to understand the mathematical thermodynamic functions first in order to input thermodynamic variables and computing unknowns that dictate what is conceptually occurring in the thermodynamic system. By applying this strategy, I was able to succeed in BMEN 290 and efficiently learn thermodynamics.
Within both my research in the Jabbarzadeh lab and BMEN 290, I learned to treat failures not only as steps closer to the final solution but also as vital learning experiences from which I utilize and tune future methodology in order optimize the final solution. Uniquely, my research project taught me to discover various other research techniques similar or even just small modifications to the techniques I have attempted in order to find the perfect solution. On the other hand, my learning experiences in BMEN 290 showed me that sometimes the correct solution is very different from what I was originally thought. Nevertheless, both of my experiences demonstrated the uniqueness of solutions and the importance of overcoming and learning from failures. In the research and engineering fields, documentation of failures is extremely critical; the documentation justifies the implementation of strategies and shows other researchers/engineers what methods have already been performed and failed so that repetition of failures can be prevented and effective solutions can be optimized.
BMEN 546 Intracellular Delivery Platform Proposal
BMEN 260 Lab Report on stress and strain analysis of materials
2018 Magellan Scholar Application for research funding through the USC Office of Undergraduate Research